As aviation sets its sights on an ambitious goal of achieving net-zero emissions by 2050, the challenges and areas of focus for Safran are in many ways a microcosm of those faced by the wider aerospace industry.
With a portfolio spanning everything from engines – commercial, military and helicopter – to landing-gears, the French aerospace giant has multiple areas requiring attention when it comes to decarbonisation.
But maturing new materials, process and designs suitable for both current and next-generation aircraft does not come cheap: last year Safran spent almost €600 million ($665 million) on research and technology (R&T) activities and that figure is set to rise in 2024. In the first half, self-funded R&T expenses were €333 million, against €262 in the same period of 2023.
Managing the effort is Eric Dalbies, Safran’s chief technology officer. He estimates that “more than three-quarters” of the company’s R&T budget is devoted to decarbonisation topics: “It is one of our big journeys”, he says.
Of course, by far “the bulk” of that spending is devoted to activities supporting the RISE commercial engine demonstrator programme being developed by its CFM International joint-venture with GE Aerospace.
Although the RISE open-fan engine is the highest profile project – one that promises fuel-burn and emissions savings of around 20% over current high-bypass turbofans – it is by no means the only one, says Dalbies.
For instance, Safran has a focus on “mass reduction everywhere we can”, he says, through both the application of advanced materials and new manufacturing techniques.
In the former case, while lightweight and heat-resistant ceramic matrix composites, or CMCs, have quickly found a place in engine hot sections, Safran is also researching the use a related material, polymer matrix composites (PMCs) for weight saving.
Although not suitable for high-temperature applications – the material is designed for a maximum of around 300°C (572°F) – PMCs are nonetheless a “real alternative for structures”, including those, such as fan or compressor casings, on engines, says Dalbies. In addition, Safran is also investigating the use of the material to replace metallic parts, such as brake rods or braces in landing-gears, for example.
“These are huge parts… if we turn to composite instead of stainless steel or titanium it is really paying off on the structure of a landing-gear; you can save kilogrammes and kilogrammes,” he says.
For a brace, the saving is in the region of 20kg (44lb) or more, he says, with the brake rods saving about 10kg each. That saving, of course, is multiplied across two sets of main landing gear per aircraft.
Those parts are at around technology readiness level (TRL) 4 or 5, but due to certification requirements, require buy-in from airframe customers before they can enter service. But acceptance of the new components depends on a host of criteria, including cost, durability, and serviceability.
“We are pushing [airframers] a collection of topics and ideas for the improvement of the aircraft,” says Dalbies, “and depending on their receptivity and relevance for aircraft optimisation… after that it sometimes takes years to get their acceptance”.
Changing the material itself “is one way to do it”, but for Safran another route to weight-saving is switching to advanced production techniques like additive layer manufacturing, or 3D printing.
At last year’s Paris air show, Safran showcased a 3D-printed hydraulic control unit for landing-gear which weighed around 10kg, against 18kg for the original cast component.
Roll-out of the new processes and materials is already ongoing, initially in the firm’s defence business, thanks to streamlined certification requirements. For example, on the M88 engine that powers the Dassault Aviation Rafale fighter, Safran has taken an engine bearing bracket, a component normally comprised of “more than 100 parts”, and “turned that, through additive manufacturing, into a single part”.
That component is “already flying”, he says, while other “less noble” parts – brackets, for instance – are also in service on civil aircraft.
Dalbies is careful not to repeat comments made by CFM’s executives on the progress being made on RISE, but he points out that the development of new materials and manufacturing processes, or hybrid-electric technologies, will contribute additional percentage points of efficiency gains.
While the open-fan architecture itself “brings the most disruptive reduction” in fuel burn, by incorporating hybridisation, and materials enabling mass reduction and higher-temperature operation “you will have lower fuel consumption for the same power”.
In addition, integration with the airframe will be more important than ever for the next generation of engines, says Dalbies.
“It can’t be taken for granted that just because you have the isolated performance for the engine you can turn this into a one-to-one performance gain at aircraft level,” says Dalbies.
“Aircraft manufacturers plan to have thin wings to reduce the drag. On the aircraft side, a significant part of the decarbonisation will come from more aggressive aerodynamic performance.” But the interaction with those wings will be different depending on the position of the engine and whether it is ducted or unducted, he notes.
Besides new engine architectures, hydrogen propulsion has for several years been touted as a route for aviation to achieve net-zero, whether as a fuel for direct combustion or used in fuel cells to generate electricity.
With a background in Safran’s space propulsion business, Dalbies is more familiar than most with hydrogen’s advantages, and the many obstacles to its use in aviation.
While he calls hydrogen a “very interesting topic”, he cautions that the “maturity of the technologies” is significantly lower than for disruptive engine designs. “There we are approaching TRL5 and plan to be at 6 by 2028, 2029. Now, if I look at hydrogen, where do we stand today? Probably TRL2. We are at TRL9 for [hydrogen] space propulsion, but we are at TRL2, 3 maximum, for the key technologies to be implemented on an aircraft,” he says.
As such, he argues that a slightly different “technology plan” is needed: “We have to take into account what are the blocking points in the technology of hydrogen that first need to be treated before considering going forward.”
Getting hydrogen to burn is not an issue, he says. “It’s about controlling the mass-flow rate, controlling the leak tightness, collecting the leaks; we know there will be leaks because the molecule is so small.
“The design principle in the space business is that you don’t have to ensure leak tightness – it’s not feasible – instead you have to make sure you collect them and remove them the right way.”
Hydrogen embrittlement – the weakening of certain materials when exposed to the element – is another challenge requiring further attention, says Dalbies, who notes that the issue is particularly acute at ambient temperatures “which is exactly what we will supply in terms of hydrogen at the entrance of an aircraft engine”.
“Even if it’s been investigated for some specific uses in aerospace propulsion, it’s a new domain to be investigated.
“So at Safran-level we have decided to build a structured plan, taking into account the fact that we are now at TRL2 and not TRL5.”
Although the size of the market potential for hydrogen is still unclear, Safran is continuing to research the topic.
“What we know is that if we don’t prepare the technology for this, we will never have a chance to be part of the game should this find a place in the market,” Dalbies says.
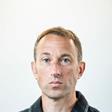